2D magnetic and topological materials
Research in magnetic thin films has revolutionized the development of spintronic devices. The search for new magnetic materials is important for improving the performance of spintronic devices. Magnetic 2D materials retain chemical stability and structural integrity of their layered bulk crystals, while at the same time can be readily tuned by various kinds of gate modulation. For these reasons, magnetic 2D materials have become a focus in spintronics research.
We are committed to exploring novel magnetic 2D materials that are potentially useful in spintronic applications. Experimentally isolating magnetic 2D materials from bulk crystals, however, poses a challenge: intralayer van der Waals bonding is often not strong enough for thin flakes of reasonable size to survive conventional mechanical exfoliation processes. Similar issues plague the exfoliation of many other layered materials (only a small fraction of known layered crystals is cleavable down to monolayers), and so have largely impeded the research on 2D materials in general.
We developed a new method, Al2O3-assisted exfoliation, which is able to produce monolayers of layered bulk materials that are otherwise difficult to cleave with conventional methods. Using Al2O3-assisted exfoliation, we obtain atomically thin Fe3GeTe2, a magnetic 2D metal. By further applying the gate-controlled lithiation technique that we developed previously, we achieve room-temperature ferromagnetism in few-layer Fe3GeTe2. This result opens up new opportunities for potential gate-controlled magneto-electronics based on 2D materials (Nature 563, 94-99, (2018)).
Novel interesting physics emerges in magnetic 2D materials with non-trivial band topology. Breaking time-reversal symmetry of 3D topological insulators (TIs) by long-range ferromagnetic order induces profound changes in their electronic structure, resulting in an exchange gap in the gapless Dirac dispersion of the surface states. The gap opening is accompanied by the emergence of a chiral edge mode that gives rise to a quantum anomalous Hall (QAH) effect when the Fermi level is situated inside the exchange gap. The dissipationless QAH edge channel, combined with the spin-momentum locking that is inherent in topological materials, may lead to novel device concepts in topological electronics. One such example is the proposed topological qubit made from the chiral fermion edge modes.
The experimental observation of the QAH effect in Cr-doped (Bi,Sb)2Te3 by Qi-Kun Xue group represents a triumph in topological quantum material research—the ratio of the multiple elements in the non-stoichiometric material has to be precisely controlled to accomplish such a feat. However, the fine-tuning needed to reconcile conflicting demands (i.e., large magnetization and low initial carrier doping) poses a challenge for material growth, and the randomly distributed magnetic dopants act as impurities that limit the quality of the magnetic TIs. Further exploration of rich topological phenomena and their potential applications calls for intrinsic magnetic TIs (stoichiometric TIs with an innate magnetic order) so that topological effects can be studied in pristine crystals.
We probe quantum transport in MnBi2Te4 thin flakes—a topological insulator with intrinsic magnetic order. In this layered van der Waals crystal, the ferromagnetic layers couple antiparallel to each other. Atomically thin MnBi2Te4, however, becomes ferromagnetic when it has an odd number of septuple layers. We observe a zero-field QAH effect in a five–septuple-layer specimen at 1.4 K, and an external magnetic field further raises the quantization temperature to 6.5 K by aligning all layers ferromagnetically. The results establish MnBi2Te4 as an ideal arena for further exploring various topological phenomena with a spontaneously broken time-reversal symmetry (Science 367, 895-900 (2020)).
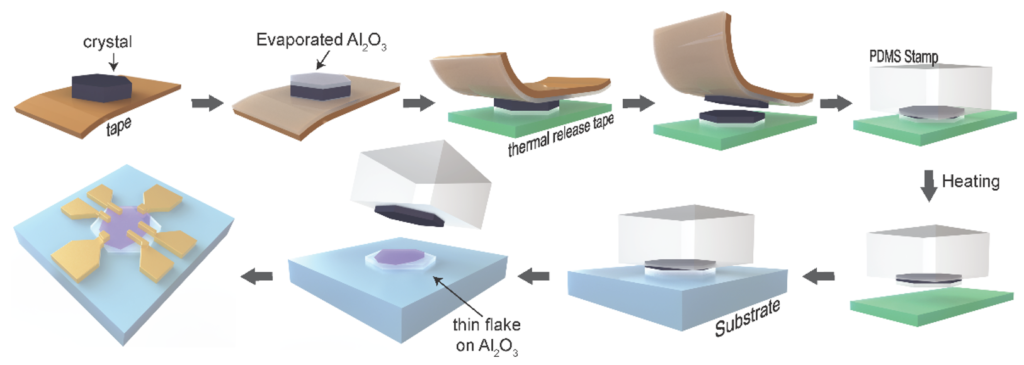
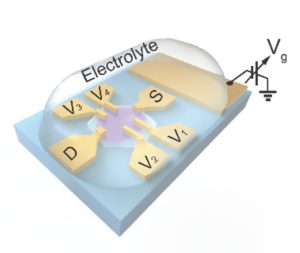
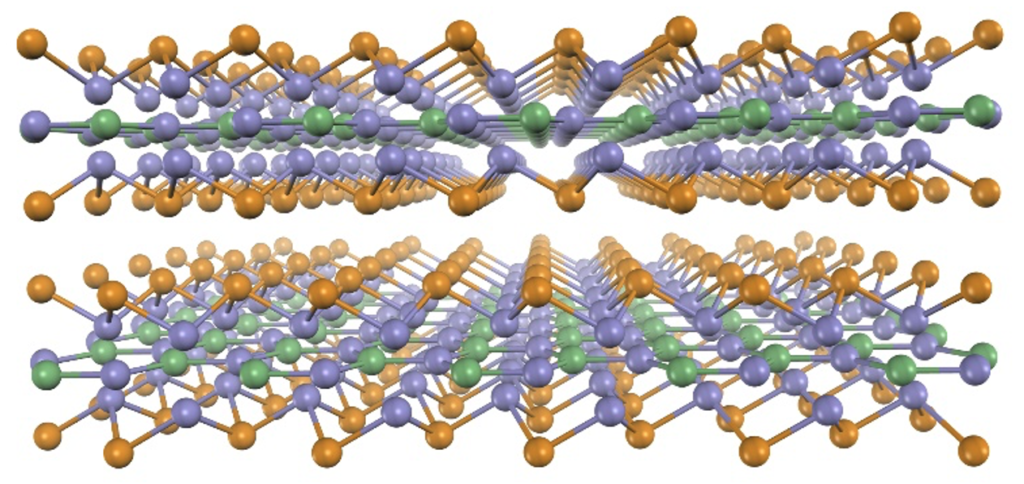